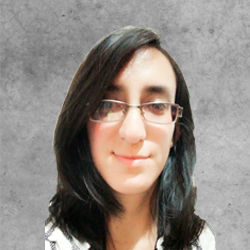
Head of Synthetic biology and Tissue Engineering Laboratory
Bioengineering and Chemical Engineering Department
3D bioprinting is a multidisciplinary area, in which life sciences and engineering converge to create tissues and organs, making use of the principles of additive manufacturing through the combination of biomaterials, cells and growth factors, which allow proper cell growth. Bioprinted tissues can address the shortage of functional tissue models for personalized medicine and drug screening.
The most commonly used types of bioprinters are: inkjet-based, laser-assisted, and extrusion-based.
Inkjet bioprinting apply a thermal, piezoelectric, or electromagnetic force to cause the release of tiny droplets of cells encapsulated in the biomaterial onto a collection plate; this method can be applied to low-viscosity biomaterials for the high-resolution deposition of thin layers and patterned structures.
Laser-assisted bioprinting is less popular since it is difficult, expensive, and difficult to utilize. Its main advantages include excellent cell survivability, retention of the majority of cell functions and morphologies, usage of a higher cell density, and avoidance of nozzle obstruction by used bioink by forgoing their utilization.
The extrusion-based printing expels the bioinks through a nozzle aided by a plunger pushed by a vacuum pump that produces the necessary pressure to produce layer-by-layer 3D constructions; which allows the use of bioinks with higher viscosity.

In space, one of the factors that is present is microgravity, which is used in bioprinting since it allows more complex geometries to be made, which would not be possible on earth due to the need for structural support due to gravity; reduces the effects of hydrodynamic shear and hydrostatic pressure, making surface tension the dominant force; causing the molecules to remain in tight spheres and films, maximizing intermolecular attraction.
Depending on the technology being used, microgravity has varying implications on the bioprinting process; Due to the slower rate at which the droplets of biomaterial fall to the surface in inkjet-based bioprinting, microgravity has an impact on droplet output and the continuous flow of biomaterial through the nozzle. Since the bioink in extrusion-based 3D bioprinters is extruded by the pneumatic system through the printer nozzle to shape a continuous filament, that shapes the final structure, so they can be used in an upward direction against Earth’s gravity, laser-assisted 3D bioprinters require thorough cleanup after the bioprinting process..
Numerous earlier research have used methods like the clinostat, random positioning machine (RPM), rotating wall vessel (RWV), and magnetic levitation to simulate microgravity on Earth.
In order to reduce the gravitational acceleration to zero, the Clinostat turns the survey system perpendicular to the gravity vector. In order to counteract the effects of gravity, random positioning machines (RPMs) rotate biological samples along two distinct axes in sophisticated ways. Magnetic levitation devices are the most popular because they do not use a mechanical rotation system, which would put additional forces and stress on the sample. Rotating Wall Vessels (RWV) are completely filled with a fluid and function similarly to clinostats by transmitting the speed of rotation to the study system.
In 2018, Rocosmos unveiled “Organ.Aut,” a magnetic bioassembler designed to investigate the potential for formative 3D biofabrication of tissue structures using the self-assembly method of living tissues and organs in microgravity. The trays they created were sealed with thermosensitive hydrogel to protect spheroids of cartilage tissue (chondrospheres) on journeys from Earth to the International Space Station. In order to trigger the thermoreversible hydrogel’s “gel-sol” transition, the paramagnetic medium was first injected into the hydrogel containing the chondrospheres. The mixture was then cooled at 17°C for 90 minutes, at which point the chondrospheres were free to move around. Six cuvettes containing the chondrospheres were united into a tissue construct after 1 hour in the magnetic field, and +37 °C was maintained for 2 days. The chondrospheres were then placed back in the soil for additional study after being fixed in 4% formaline and stored at room temperature for 2 weeks. The chondrocytes were able to sustain their vitality and biochemical activity, similar to 3D cultures in soil, according to analysis of the 3D tissue construct.

In 2019, the BioFabrication Facility (BFF) was launched together with the Techshot bioreactor cassettes from the ISS National Lab, using extrusion as the bioprinting technique. The cassettes will store the tissue produced. For the bioprinting process, they designed a platform with linear motors with movement in the three axes applied to the print head. This system had a resolution of 100 nm, a repeatability of 500 nm and a precision of 1 μm. The first experiment wanted to generate a structure similar to heart tissue, for which 100 layers of bioink were dispensed, resulting in a build volume of ≈19.3 cm3.

The main challenges that bioprinting in space has is the high cost of the logistics necessary for packaging and transportation (the cost can vary from 4,100 USD to 2,700,000 USD per kilogram of weight) so that the samples arrive viable at the ISS. , they must also ensure the safety of the astronauts who use the devices, so the hoses or any device where the passage of liquids occurs must be leak-proof.
In order for biological samples to remain alive, different pre-print and post-print storage temperatures are needed until arrival in the soil for cellular and molecular viability studies.
Electronic components and biological samples must be shielded from radiation, since the perceived level of ionizing radiation equivalent to 6 months on the ISS is 80 mSv.
Conclusions
Due to microgravity, it is possible to bioprint structures employing more fluidic systems and, thus, more biocompatible bioinks; ,because there is no gravity, complex geometries like voids, holes, and tunnels can be constructed more easily in microgravity because they are self-supported there rather than on Earth.
Bibliography
- Tabury, K., Rehnberg, E., Baselet, B., Baatout, S., & Moroni, L. (2023). Bioprinting of Cardiac Tissue in Space : Where Are We ? 2203338, 1–15. https://doi.org/10.1002/adhm.202203338
- Rezapour Sarabi, M., Yetisen, A. K., & Tasoglu, S. (2023). Bioprinting in Microgravity. ACS Biomaterials Science and Engineering. https://doi.org/10.1021/acsbiomaterials.3c00195
- Parfenov, V. A., Petrov, S. V., Pereira, F. D. A. S., Levin, A. A., Koudan, E. V., Nezhurina, E. K., Karalkin, P. A., Vasiliev, M. M., Petrov, O. F., Komlev, V. S., Khesuani, Y. D., & Mironov, V. A. (2020). Scaffold-free, label-free, and nozzle-free magnetic levitational bioassembler for rapid formative biofabrication of 3D tissues and organs. International Journal of Bioprinting, 6(3), 110–119. https://doi.org/10.18063/IJB.V6I3.304